
THE
HEAT
PRODUCTION
OF
FATIGUE
AND
ITS
RELATION
TO
THE
PRODUCTION
OF
LACTIC
ACID
IN
AMPHIBIAN
MUSCLE.
BY
RUDOLPH
A.
PETERS,
Benn
Levy
Student
and
Student
of
Gonville
and
Caius
College.
(From
the
Phy
iological
Laboratory,
Camrtidge.)
Introduction.
The
belief
that
the
contraction
of
a
muscle
is
due
primarily
to
the
liberation
of
lactic
acid
from
some
precursor
at
or
near
some
surface
in
the
fibril,
so
as
to
cause
a
rise
of
tension
in
the
muscle
has
gained
support
from
several
recent
investigations.
That
the
shortening
of
muscle
in
fatigue
and
rigor
is
due
to
the
action
of
metabolic
products,
chiefly
of
lactic
acid,
has
been
a
view
in
existence
from
the
time
of
Rankel;
and
it
has
been
suggested
by
Gad2
that
the
normal
contraction
has
a
similar
causation.
It
has
been
brought
into
prominence
again
owing
to
the
work
of
Fletcher
and
Hopkins8.
In
their
work
they
showed
that
fatigue
was
associated
with
a
marked
increase
of
lactic
acid,
and
that
the
process
culminating
in
the
stiffening
of
rigor
was
accompanied
by
a
certain
maximum
production
of
acid.
Again
A.
V.
Hill
has
argued
from
the
production
of
heat
and
its
relation
to
the
time
of
the
mechanical
response
that
the
contraction
probably
takes
place
in
this
manner.
Considering
the
summation
of
contractions,
Mines5
has
pointed
out
that
a
sudden
liberation
of
acid
might
certainly
be
the
cause
of
the
electric
change,
which
he
shows6
for
his
combined
curves
of
the
electrical
and
mechanical
response
in
the
excised
frog's
heart
to
occur
before
the
mechanical
response.
That
acid
liberated
upon
the
surface
of
some
colloidal
structures
will
produce
a
shortening
is
known
from
the
work
of
Fischer
and
Strietman7.
Acid
rigor
has
1R
anke.
Grundziig
der
Phys.
p.
632.
2
Gad.
Arch.
f.
Phys.
p.
166.
1893.
3
Fletcher
and
Hopkins.
This
Journal,
xxxv.
p.
247.
1907.
4
A.
V.
Hill.
Ibid.
xrvi.
p.
28.
1913.
6
Mines.
Ibid.
xLVI.
p.
1.
1913.
6
Mines.
Ibid.
XLVI.
p.
188.
7
Fischer
and
Strietman.
Koll.
Zeit.
x.
1912.

R.
A.
PETERS.
long
been
known
and
Fl
etcher'
has
given
curves
showing
the
shortening
of
a
muscle
in
lactic
acid.
Upon
a
priori
grounds,
it
might
be
supposed
that
the
liberation
of
a
quantity
of
lactic
acid
sufficiently
large
to
cause
the
rise
of
tension
observed
in
a
muscle
would
be
attended
by
some
thermal
change
as
well
as
by
an
electrical
one.
It
has
been
long
known
that
a
thermal
change
existed
in
contraction,
and
A.
V.
Hill2
has
estimated
the
heat
evolved
under
the
same
conditions
as
the
lactic
acid
production
observed
by
Fletcher
and
Hopkins.
Hill
shows
that
the
heat
produced
during
the
survival
life
of
amphibian
muscle
follows
approximately
the
curves
of
CO
and
of
lactic
acid
production.
All
conditions
which
affect
the
liberation
of
lactic
acid
seem
to
affect
the
evolution
of
heat
in
a
similar
sense,
there
being
for
heat
the
same
attainment
of
a
maximum
in
heat
and
chloroform
rigor
as
for
lactic
acid.
In
one
case,
however,
Hill
did
not
study
the
simultaneous
production
of
heat
and
lactic
acid,
the
production
of
heat
during
the
fatigue
induced
by
artificial
stimulation.
It
is
known
from
the
work
of
Fletcher
and
Hopkins
that
acid
is
formed
when
fatigue
is
induced
in
this
manner.
After
severe
and
prolonged
stimulation,
they
obtained
a
yield
of
about
half
the
amount
of
acid
to
be
obtained
in
complete'
rigor,
either
by
heat
or
chloroform,
being
the
same
approximately
for
both.
This
was
obtained
usually
by
direct
stimulation;
in
one
case
where
indirect
was
employed,
a
similar
result
was
obtained.
Fatigue
was
shown
by
Hill
to
reduce
the
heat
production
of
chloroform
rigor
in
the
same
sense
that
it
was
reduced
by
periods
of
survival
life.
This
reduction
seemed
to
give
further
grounds
for
believing
that
rigor
was
an
extended
form
of
the
process
of
contraction,
that
in
both
cases
there
was
the
liberation
of
chemical
bodies,
with
the
difference
that
in
rigor
the
liberation
(or
breakdown)
went
further.
Such
a
view
has
been
held
by
Nysten
(1811),
by
Schiff3
and
by
Hermannt.
By
a
better
method
Fletcher5
confirmed
the
latter's
demonstration
that
the
CO,
yielded
in
heat
rigor
was
diminished
by
previous
fatigue
with
the
circulation
intact,-i.e.
that
the
processes
of
rigor
and
contraction
made
calls
upon
the
same
precursor.
If
the
processes
which
are
the
immediate
cause
of
contraction
are
1
Fletcher.
This
Journal,
xIzzib
p.
54.
1898.
2
A.
V.
Eill.
Ibid.
xL.
p.
466.
1912.
3
Schiff.
Beitr.
z.
Physiol.
a.
p.
9.
1894.
4
Hermann.
Stoffwechsel
der
Muskel.
1867.
5
Fletcher.
This
Journal,
xxii.
p.
10.
1898.
244

HEAT
PRODUCTION
OF
MUSCLE.
the
same
as
those
which
lead
ultimately
to
rigor
it
is
not
unreasonable
to
suppose
that
the
amount
of
heat
liberated
during
a
given
degree
of
fatigue
should
bear
to
the
heat
production
of
chloroform
rigor,
the
same
relation
as
the
lactic
acid
produced
during
a
similar
period
bears
to
the
total
acid
maximum.
For
if
the
processes
of
contraction
involved
other
reactions
(such
e.g.
as
oxidations)
than
those
culminating
in
rigor,
the
heat
production
of
contraction
might
be
incomparably
larger
in
proportion.
Therefore
it
seemed
that
an
opportunity
was
given
of
testing
the
hypothesis
-
outlined
above,
as
well
as
establishing
any
relation
that
might
exist
between
heat
production
and
the
appearance
of
lactic
acid.
For
if
the
heat
production
of
chloroform
rigor
in
the
fresh
muscle
was
roughly
equal
to
the
heat
produced
during
stimulation
plus
the
heat
production
of
chloroform
rigor
after
stimulation,
it
would
not
be
necessary
to
assume
that
any
process
had
occurred
during
stimulation
other
than
that
which
had
occurred
in
the
process
leading
to
rigor.
(Any
other
change
that
might
occur
would
necessarily
be
one
taking
place
with
small
evolution
or
absorption
of
heat,
and
therefore
probably
of
comparatively
small
importance.)
If
on
the
other
hand
the
heat
produced
during
prolonged
contraction
plus
the
heat
production
of
chloroform
rigor
after
contraction
was
much
greater
or
much
less
than
the
heat
production
of
chloroform
rigor
in
the
fresh
muscle,
another
exothermic
or
endothermic
process
would
be
occurring
during
the
contraction,
and
the
hypothesis
would
need
modification.
In
this
paper
the
question
has
been
investigated
from
the
standpoint
of
the
following
main
questions.
(1)
Was
the
maximum
heat
production
of
fatigue
definitely
greater
than
the
heat
production
of
chloroform
rigor
in
the
fresh
muscle?
(2)
If
not,
was
the
maximum
heat
liberated
during
contraction
plus
that
liberated
during
chloroform
rigor
after
fatigue
approximately
equal
to
the
heat
production
of
chloroform
rigor
in
the
fresh
muscle,
and
what
effect
did
the
previous
saturation
of
the
tissue
with
oxygen
have
upon
this
equality?
(3)
Further
was
the
relation
between
the
mnaximum
heat
produc-
tion
of
a
muscle
contracting
to
a
condition
of
fatigue
and
that
of
chloroform
rigor
in
the
fresh
muscle
of
the
same
order
as
that
between
the
amounts
of
lactic
acid
liberated
under
similar
circumstances?
If
so,
there
would
be
further
grounds
for
believingin
an
extremely
intimate
connection
between
the
lactic
acid
appearing
in
a
muscle
and
the
evolution
of
heat.
245
16
PH.
XLVII.
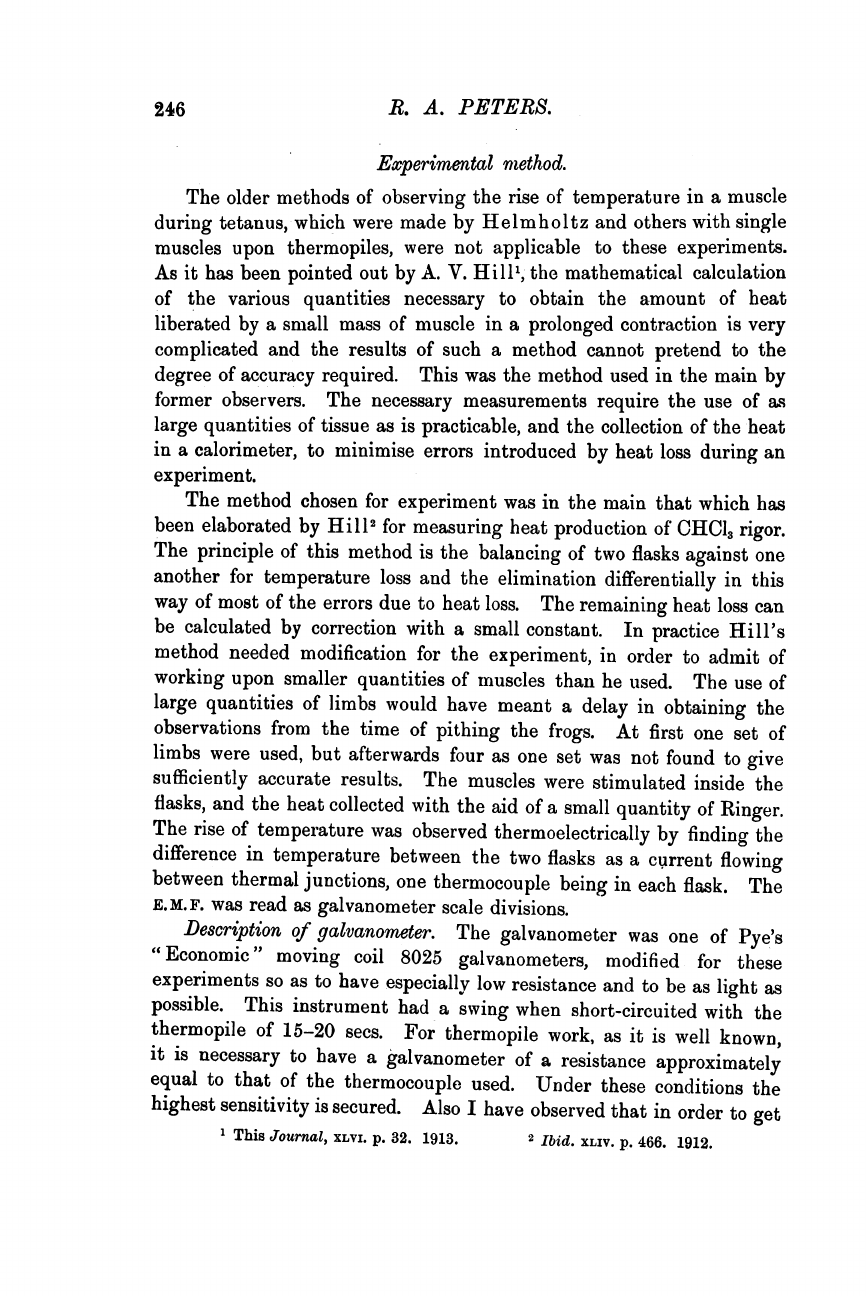
R.
A.
PETERS.
Experimental
method.
The
older
methods
of
observing
the
rise
of
temperature
in
a
muscle
during
tetanus,
which
were
made
by
Helmholtz
and
others
with
single
muscles
upon
thermopiles,
were
not
applicable
to
these
experiments.
As
it
has
been
pointed
out
by
A.
V.
Hilll,
the
mathematical
calculation
of
the
various
quantities
necessary
to
obtain
the
amount
of
heat
liberated
by
a
small
mass
of
muscle
in
a
prolonged
contraction
is
very
complicated
and
the
results
of
such
a
method
cannot
pretend
to
the
degree
of
accuracy
required.
This
was
the
method
used
in
the
main
by
former
observers.
The
necessary
measurements
require
the
use
of
as
large
quantities
of
tissue
as
is
practicable,
and
the
collection
of
the
heat
in
a
calorimeter,
to
minimise
errors
introduced
by
heat
loss
during
an
experiment.
The
method
chosen
for
experiment
was
in
the
main
that
which
has
been
elaborated
by
Hill2
for
measuring
heat
production
of
CHC003
rigor.
The
principle
of
this
method
is
the
balancing
of
two
flasks
against
one
another
for
temperature
loss
and
the
elimination
differentially
in
this
way
of
most
of
the
errors
due
to
heat
loss.
The
remaining
heat
loss
can
be
calculated
by
correction
with
a
small
constant.
In
practice
Hill's
method
needed
modification
for
the
experiment,
in
order
to
admit
of
working
upon
smaller
quantities
of
muscles
than
he
used.
The
use
of
large
quantities
of
limbs
would
have
meant
a
delay
in
obtaining
the
observations
from
the
time
of
pithing
the
frogs.
At
first
one
set
of
limbs
were
used,
but
afterwards
four
as
one
set
was
not
found
to
give
sufficiently
accurate
results.
The
muscles
were
stimulated
inside
the
flasks,
and
the
heat
collected
with
the
aid
of
a
small
quantity
of
Ringer.
The
rise
of
temperature
was
observed
thermoelectrically
by
finding
the
difference
in
temperature
between
the
two
flasks
as
a
current
flowing
between
thermal
junctions,
one
thermocouple
being
in
each
flask.
The
E.M.F.
was
read
as
galvanometer
scale
divisions.
Description
of
galvanometer.
The
galvanometer
was
one
of
Pye's
"Economic
"
moving
coil
8025
galvanometers,
modified
for
these
experiments
so
as
to
have
especially
low
resistance
and
to
be
as
light
as
possible.
This
instrument
had
a
swing
when
short-circuited
with
the
thermopile
of
15-20
secs.
For
thermopile
work,
as
it
is
well
known,
it
is
necessary
to
have
a
galvanometer
of
a
resistance
approximately
equal
to
that
of
the
thermocouple
used.
Under
these
conditions
the
highest
sensitivity
is
secured.
Also
I
have
observed
that
in
order
to
get
1
This
Journal,
XLVI.
p.
32.
1913.
2
Ibid.
XLIV.
p.
466.
1912.
246
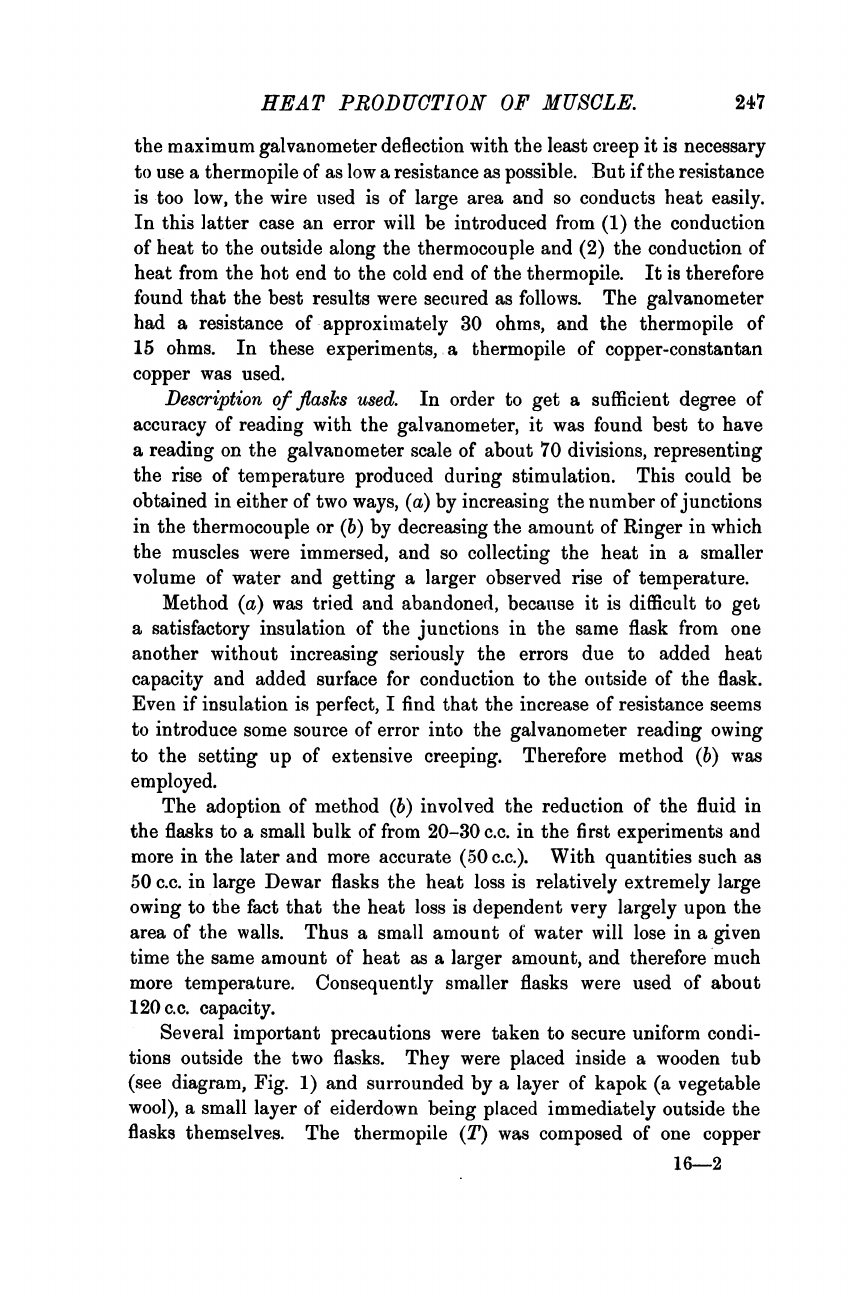
HEAT
PRODUCTION
OF
MUSCLE.
the
maximum
galvanometer
deflection
with
the
least
creep
it
is
necessary
to
use
a
thermopile
of
as
low
a
resistance
as
possible.
But
if
the
resistance
is
-too
low,
the
wire
used
is
of
large
area
and
so
conducts
heat
easily.
In
this
latter
case
an
error
will
be
introduced
from
(1)
the
conduction
of
heat
to
the
outside
along
the
thermocouple
and
(2)
the
conduction
of
heat
from
the
hot
end
to
the
cold
end
of
the
thermopile.
It
is
therefore
found
that
the
best
results
were
secured
as
follows.
The
galvanometer
had
a
resistance
of
-approximately
30
ohms,
and
the
thermopile
of
15
ohms.
In
these
experiments,
a
thermopile
of
copper-constantan
copper
was
used.
Description
of
flasks
used.
In
order
to
get
a
sufficient
degree
of
accuracy
of
reading
with
the
galvanometer,
it
was
found
best
to
have
a
reading
on
the
galvanometer
scale
of
about
70
divisions,
representing
the
rise
of
temperature
produced
during
stimulation.
This
could
be
obtained
in
either
of
two
ways,
(a)
by
increasing
the
number
of
junctions
in
the
thermocouple
or
(b)
by
decreasing
the
amount
of
Ringer
in
which
the
muscles
were
immersed,
and
so
collecting
the
heat
in
a
smaller
volume
of
water
and
getting
a
larger
observed
rise
of
temperature.
Method
(a)
was
tried
and
abandoned,
becauise
it
is
difficult
to
get
a
satisfactory
insulation
of
the
junctions
in
the
same
flask
from
one
another
without
increasing
seriously
the
errors
due
to
added
heat
capacity
and
added
surface
for
conduction
to
the
ouitside
of
the
flask.
Even
if
insulation
is
perfect,
I
find
that
the
increase
of
resistance
seems
to
introduce
some
source
of
error
into
the
galvanometer
reading
owing
to
the
setting
up
of
extensive
creeping.
Therefore
method
(b)
was
employed.
The
adoption
of
method
(b)
involved
the
reduction
of
the
fluid
in
the
flasks
to
a
small
bulk
of
from
20-30
c.c.
in
the
first
experiments
and
more
in
the
later
and
more
accurate
(50
c.c.).
With
quantities
such
as
50
c.c.
in
large
Dewar
flasks
the
heat
loss
is
relatively
extremely
large
owing
to
the
fact
that
the
heat
loss
is
dependent
very
largely
upon
the
area
of
the
walls.
Thus
a
small
amount
of
water
will
lose
in
a
given
time
the
same
amount
of
heat
as
a
larger
amount,
and
therefore
much
more
temperature.
Consequently
smaller
flasks
were
used
of
about
120
c.c.
capacity.
Several
important
precautions
were
taken
to
secure
uniform
condi-
tions
outside
the
two
flasks.
They
were
placed
inside
a
wooden
tub
(see
diagram,
Fig.
1)
and
surrounded
by
a
layer
of
kapok
(a
vegetable
wool),
a
small
layer
of
eiderdown
being
placed
immediately
outside
the
flasks
themselves.
The
thermopile
(T)
was
composed
of
one
copper
16-2
247

B.
A.
PETERS.
constantan
junction
in
each
flask.
One
piece
of
constantan
wire
was
passed
fromn
the
bottom
of
one
flask
to
the
bottom
of
the
other
insulated
in
glass
tubing;
to
each
end
of
the
constantan,
copper
wires
were
fused
and
passed
from
the
flasks
as
leads
(T')
to
the
galvanometer.
Insulation
was
carried
out
by
enclosing
the
wires
in
fine
glass
tubes;
the
ends
of
these
which
dipped
in
the
fluid
were
sealed
by
binding
with
fine
indiarubber
membrane.
This
method
of
enclosing
in
fine
glass
tubes
gave
the
additional
advantage
that
the
thermo-junctions
were
kept
rigidly
in
the
bottom
of
the
flasks.
This
was
found
to
be
extremely
~~~iIA.~
~~.
£
7
7
thog
idedw
ad
kao
oteousd
fte
odntb
Lfo'
ib
T
//
T
k
-
---V.
L
Fig.
1.
Calorimeter
used
in
experiments
upon
heat
produced
during
stimulation.
V=vacuum.
flask.
T=thermopile
with
T'
copper
leads
to
galvanometer
passing
through
eiderdown
and
kap'ok
to
the
outside
of
the
wooden
tub.
L=frog's
limbs
attached
to
stimulating
apparatus
for
enlarged
diagram
of
which
see
Figs.
2a
and
2
b.
W=wires
for
stimulation,
passing
through
fine
glass
rod
to
the
ebonite
disc
to
which
the
muscles
are
attached.
A.
S.
=
apparatus
for
stirring
automatically
both
glass
rods,
lifting
them
equal
heights.
important
when
dealing
with
these
small
quantities
of
fluid
in
the
bottom
of
the
flasks,
because
if
the
thermo-junction
were
not
covered,
serious
errors
of
reading
were
found
to
occur.
The
flasks
were
cemented
together
with
a
small
piece
of
wood
between
them
to
prevent
direct
contact
and
the
thermopile
cemented
into
position
in
the
flasks.
In
this
way
the
apparatus
was
made
stable.
The
copper
leads
from
the
ends
of
the
thermopile
were
brought
to
the
outside
of
the
tub
and
248

HEAT
PRODUCTION
OF
MUSCLE.
taken
direct
into
the
mercury
of
a
Pohl
commutator,
which
was
connected
with
the
galvanometer.
Cylinders
of
American
cloth
were
then
made
which
fitted
exactly
the
mouths
of
the
flasks,
and
these
were
cemented
into
the
mouths
of
the
flasks
so
that
water
poi'red
inside
the
flasks
could
not
leak
into
the
interior
of
the
tub.
Holes
were
bored
in
the
lid
of
the
tub
into
which
were
fitted
large
pieces
of
glass
tube
of
such
a
size
that
the
cloth
cylinders
would
just
fit
inside
them;
by
sinking
the
flasks
completely
beneath
the
wool,
it
was
arranged
that
the
only
place
for
conduction
of
heat
to
take
place
should
be
by
the
cloth
cylinder.
Corks
were
fitted
to
the
glass
tubes,
which
were
bored
and
fitted
with
small
glass
tubes
to
take
the
glass
rod
to
which
the
muscles
were
attached.
In
the
construction
of
this
apparatus
I
have
to
express
my
thanks
to
Dr
C.
G.
L.
Wolf
for
his
kind
help.
Apparatus
for
stimulating
the
muscles.
In
the
first
experiments
before
the
foregoing
apparatus
was
constructed,
it
was
attempted
to
stimulate
the
muscles
directly.
This
plan
was
abandoned,
however,
in
favour
of
indirect
stimulation;
some
justification
for
this
abandonment
was
that
Fletcher
and
Hopkins
found
that
they
obtained
the
same
lactic
acid
yield
whether
the
stimulation
was
direct
through
the
muscles
or
indirect
through
the
nerves;
they
performed
however
only
one
experiment
upon
this
point.
Indirect
stimulation
had
the
following
great
advantage
that
it
was
necessary
to
use
only
an
extremely
weak
current,
which
produced
a
heat
production
of
its
owin
practically
negligible.
The
problem;
then,
narrowed
itself
into
fitting
4-6
limb
pairs
into
the
flasks
in
such
a
way
that
the
limbs
were
immersed
in
Ringer,
were
attached
to
a
glass
rod
which
should
admit
of
stirring
and
through
which
could
pass
wires
for
stimulating
the
nerves.
The
whole
bad
to
be
of
as
little
heat
capacity
as
possible
and
to
present
as
little
opportunity
for
the
leakage
of
heat
to
or
from
the
exterior
according
as
the
temperature
of
the
room
was
above
or
below
that
of
the
interior
of
the
flask.
This
was
finally
done
as
follows;
for
diagram
see
Fig.
2
a
and
2
b.
A
circle
of
ebonite
of
radius
33
mm.
and
depth
8
mm.
was
hollowed
out
in
the
centre
so
as
to
form
a
circular
groove
with
the
exception
of
a
small
boss,
which
was
left
and
drilled
centrally
to
hold
exactly
the
glass
rod
containing
the
stimulating
wires.
In
the
narrow
margin
left
round
the
edge
were
drilled
six
pairs
of
two
holes
at
such
a
distance
apart
that
they
were
fitted
exactly
to
receive
the
two
bones
forming
249

R.
A.
PETERS.
the
pelvic
girdle
of
the
frog's
limbs.
(This
was
found
to
be
7
mm.)
Between
each
of
these
pairs
of
holes
was
cut
a
small
slit
extending
into
the
circular
trough
1
mm.
deep
and
1
mm.
in
cross
section
at
the
place
where
it
entered
the
trough,
being
widened
as
it
reached
the
outside
and
extended
down
between
the
two
boles
designed
to
hold
the
pelvic
bones.
A
small
horizontal
groove
was
also
cut
in
the
boss
in
the
centre.
The
finest
glass
tube
obtainable
was
then
fitted
with
the
stimulating
wires
as
follows.
A
hole
was
blown
in
the
side
of
the
tube
and
a
piece
of
platinum
wire
to
which
was
attached
fine
copper
wire
(No.
38),
fuised
into
the
side
leaving
as
little
prominence
as
possible.
A
similar
wire
was
then
Fig.
2a.
Fig.
2b.
Fig.
2
a.
Enlarged
view
of
ebonite
discs
with
muscles
attached.
The
nerves
from
the
muscles
are
indicated
passing
through
the
1
mm.
square
groove
into
the
central
trough.
The
glass
rod
down
which
the
stimulating
wires
pass
is
also
shown.
Fig.
2b.
Sectional
view
of
Fig.
2a,
showing
positions
of
electrodes,
one
inside
trough,
and
one
passing
through
end
of
glass
below
the
plate.
The
electrodes
are
platinum,
and
are
fused
into
the
glass
tube.
fitted
in
the
bottom
of
the
tube,
and
the
inside
of
the
tube
filled
with
paraffin
for
insulation
before
sealing.
The
hole
in
the
ebonite
plate
was
drilled
to
fit
the
glass
exactly.
The
side
electrode
then
was
coiled
inside
the
trough
and
the
other
below
the
ebonite.
Lastly
a
plate
of
thin
ebonite
was
arraDged
to
fit
closely
upon
the
top
of
the
trough
so
that
the
only
passage
of
the
current
from
inside
to
outside
electrode
was
through
the
six
boles
all
bored
accurately
to
the
same
size.
250

HEAT
PRODUCTION
OF
MUSCLE.
Through
these
holes
passed
the
nerves,
the
ends
attached
to
the
spinal
column
lying
inside
the
trough.
When
the
limbs
were
in
position
and
the
ebonite
plate
firmly
fitting
over
the
circular
trough,
the
stimulating
current
passed
from
the
platinum
coil
in
the
trough,
through
the
holes
containing
the
nerves,
through
the
Ringer
to
the
platinum
coil
below
the
ebonite
disc.
This
is
the
principle
employed
by
Lucas
in
stimulation,
the
stimulation
being
limited
to
the
abrupt
change
of
current
density
at
the
grooves
through
which
the
nerves
passed.
I
am
deeply
indebted
to
Dr
L
ucas
for
help
in
the
designing
of
this
apparatus,
and
for
cutting
out
the
ebonite
trough
for
me
himself.
Apparatus
for
stirring.
(Fig.
1,
A.
S.)
In
the
earliest
experiments
the
stirring
apparatus
was
left
uncontrolled,
but
this
was
found
to
produce
an
error
of
considerable
size
where
such
small
quantities
of
heat
were
being
observed.
It
was
therefore
necessary
to
control
this,
which
was
done
by
stirring
two
similar
sets
of
muscles
one
set
in
each
flask
an
equal
amount.
This
was
done
quite
simply
as
follows:-Two
upright
posts
were
fitted
across
the
openings
of
the
flasks
which
were
arranged
to
hold
a
crossbar
of
brass
upon
which
stood
a
small
brass
pulley.
Over
this
pulley
ran
a
cord
attacbed
to
the
excentric
of
a
snmall
motor,
the
end
of
the
cord
had
a
small
hook
attached
to
it,
and
this
fitted
over
a
small
bent
glass
rod
(V-shaped).
The
lower
ends
of
the
glass
rod
were
attached
to
two
hooks
cemented
to
the
upper
part
of
the
rods
which
held
the
muscles.
By
means
of
this
apparatus,
one
stir
of
the
motor
or
as
many
as
were
required
could
be
made
equally
in
each
flask.
The
control
for
the
stirring
was
tested
several
times,
by
giving
200
stirs
and
observing
whether
any
change
could
be
observed
in
the
shape
of
the
ordinary
cooling
curve.
It
was
always
found
that
there
was
no
difference
to
be
detected.
In
practice,
20
stirs
were
given
between
each
set
of
readings.
The
additional
advantage
of
the
above
apparatus
was
that
it
was
never
necessary
to
approach
the
calorimeter
and
to
introduce
irregularities
due
to
radiation
from
the
body.
0
Avoidance
of
thermoelectric
errors
in
external
circuits.
These
were
made
as
simple
as
possible,
and
mainly
of
copper
to
avoid
thermoelectric
errors
in
the
circuit.
The
copper
leads
fronm
the
thermopile
were
fused
to
thick
copper
wires,
which
passed
straight
to
the
mercury
cups
of
a
Pohl
commutator,
into
which
also
were
brought
the
galvanometer
leads.
Hence,
the
thermoelectric
effects
could
be
obtained
except
for
the
junctions
in
the
flasks
only
at
(1)
the
copper-mercury
connections
of
the
commutator;
(2)
the
galvanometer
terminals.
The
effect$
at
(1)
were
minimised
by
arranging
a
bent
glass
rod
to
work
the
tip
key
of
251
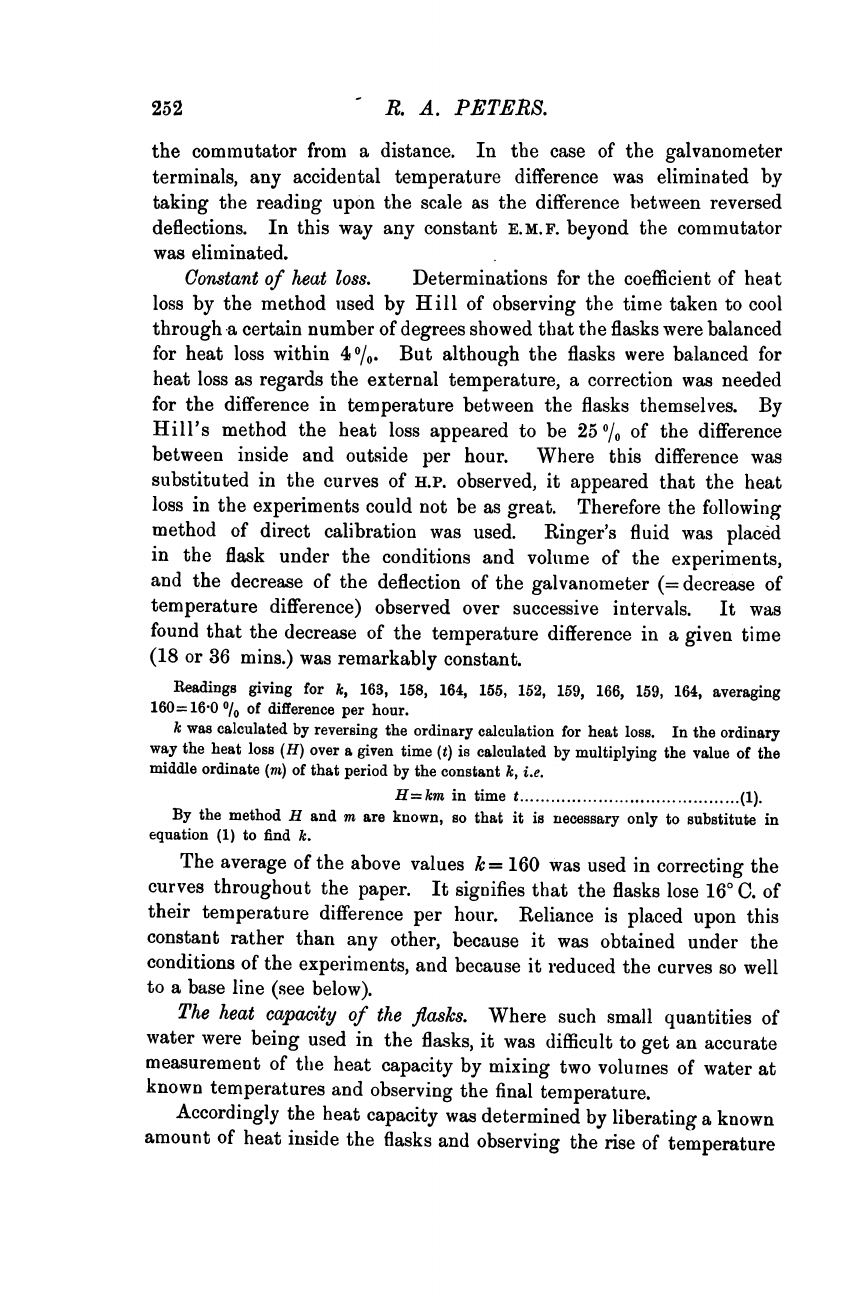
2
R.
A.
PETERS.
the
commutator
from
a
distance.
In
the
case
of
the
galvanometer
terminals,
any
accidental
temperature
difference
was
eliminated
by
taking
the
reading
upon
the
scale
as
the
difference
between
reversed
deflections.
In
this
way
any
constant
E.M.F.
beyond
the
commutator
was
eliminated.
Constant
of
heat
loss.
Determinations
for
the
coefficienit
of
heat
loss
by
the
method
uised
by
Hill
of
observing
the
time
taken
to
cool
through
a
certain
number
of
degrees
showed
that
the
flasks
were
balanced
for
heat
loss
within
40/0.
But
although
the
flasks
were
balanced
for
heat
loss
as
regards
the
external
temperature,
a
correction
was
needed
for
the
difference
in
temperature
between
the
flasks
themselves.
By
Hill's
method
the
heat
loss
appeared
to
be
25
0/0
of
the
difference
between
inside
and
outside
per
hour.
Where
this
difference
was
substituted
in
the
curves
of
H.P.
observed,
it
appeared
that
the
heat
loss
in
the
experiments
could
not
be
as
great.
Therefore
the
following
method
of
direct
calibration
was
used.
Ringer's
fluid
was
placed
in
the
flask
under
the
conditions
and
voluime
of
the
experiments,
and
the
decrease
of
the
deflection
of
the
galvanometer
(=
decrease
of
temperature
difference)
observed
over
successive
intervals.
It
was
found
that
the
decrease
of
the
temperature
difference
in
a
given
time
(18
or
36
mins.)
was
remarkably
constant.
Readings
giving
for
k,
163,
158,
164,
155,
152,
159,
166,
159,
164,
averaging
160=
16'0
0/0
of
difference
per
hour.
k
was
calculated
by
reversing
the
ordinary
calculation
for
heat
loss.
In
the
ordinary
way
the
heat
loss
(H)
over
a
given
time
(t)
is
calculated
by
multiplying
the
value
of
the
middle
ordinate
(m)
of
that
period
by
the
constant
k,
i.e.
H=km
in
time
t
...........
(1).
By
the
method
H
and
m
are
known,
so
that
it
is
necessary
only
to
substitute
in
equation
(1)
to
find
k.
The
average
of
the
above
values
k=
160
was
used
in
correcting
the
curves
throughout
the
paper.
It
signifies
that
the
flasks
lose
160
C.
of
their
temperature
difference
per
hour.
Reliance
is
placed
upon
this
constant
rather
than
any
other,
because
it
was
obtained
under
the
conditions
of
the
experiments,
and
because
it
reduced
the
curves
so
well
to
a
base
line
(see
below).
The
heat
capacity
of
the
flasks.
Where
such
small
quantities
of
water
were
being
used
in
the
flasks,
it
was
(lifficult
to
get
an
accurate
measurement
of
the
heat
capacity
by
mixing
two
volumnes
of
water
at
known
temperatures
and
observing
the
final
temperature.
Accordingly
the
heat
capacity
was
determined
by
liberating
a
known
amount
of
heat
inside
the
flasks
and
observing
the
rise
of
temperature
252

HEAT
PRODUCTION
OF
MUSCLE.
253
produced
in
a
given
volume
of
water
as
a
deflection
upon
the
galvano-
meter
scale.
This
method
proved
to
be
useful
also
for
checking
the
working
of
the
apparatus.
Heat
was
liberated
by
passing
a
known
current
for
a
known
time
through
a
coil
of
constantan
wire
of
known
resistance
wrapped
round
a
piece
of
vulcanite
of
the
same
size
as
that
used
for
holding
the
muscles.
To
do
this,
the
coil
of
wire
was
connected
with
a
two
volt
accumulator,
a
key
being
placed
in
the
circuit.
The
voltage
was
read
from
a
sensitive
voltmeter
connected
in
parallel
with
the
resistance.
The
amount
of
heat
liberated
can
be
obtained
from
the
following
formula
(Joule).
£2
R-r
Heat
liberated
(in
coil
in
flask)
E.
R
X
where
R=resistance
of
coil
immersed
in
water+resistance
of
leads
from
accumulator,
and
r
=resistance
of
leads
from
accumulator.
The
coil
used
for
the
calibration
had
a
resistance
with
the
leads
of
5-383
ohms,
the
leads
having
resistance
0-0233
ohm.
£2
5-360
Whence
H=
j-x
(538t)
In
a
given
case,
E=
1-981
volts.
Time=
179*8
sees.
H=
(1.981)2
X5x360
179-8=31-2
calories.
4,18
x
(5.383)2-
The
galvanometer
deflection
in
scale
divisions
=144,
corresponding
to
a
rise
of
1440
C=
434
C
332
Hence
volume
of
water
(60
c.0.)
in
flask+
water
equivalent
of
flask
have
been
raised
.4340
C.
by
312,
i.e.
10
C.
by
34-2=72
cals.
Since
1
cal.
raises
1
c.c.
of
water
10
C.,
eliminating
60
c.c.
due
to
water
in
flask,
we
obtain
Heat
capacity=72
-
60=
12
c.c.
Hf20.
By
this
means,
for
the
heat
aapacity
of
flask
were
found
the
following
values:
15,
11,
11,
17,
17.
Av.
14.
18,
14,
12,
12,
12.
Av.
13-6.
Average
taken
as
14
c.c.
The
numbers
are
extremely
variable,
but
the
method
is
liable
to
considerable
errors,
owing
to
difficulty
of
reading
the
small
voltage.
Approximate
accuracy
only
can
be
claimed
for
the
average
14
c.c.
A
difference
of
5
c.c.
on
either
side
would
not
make
any
serious
difference
in
the
calculated
results.
Strength
of
current
employed
for
8timulation
and
avoidance
of
the
Wedenlsky
effect.
In
the
primary
circuit
of
an
ordinary
induction
coil
were
used
two
dry
batteries
giving
three
volts.
The
position
of
the
secondary
coil
was
roughly
calibrated
so
as
to
give
the
approximate

R.
A.
PETERS.
current
strengths
for
different
positions
or
distances
from
the
primary
coil.
At
first
the
ordinary
tetanising
hammer
was
employed,
but
this
was
discarded
later
as
it
was
found
to
have
an
extremely
rapid
rate
of
stimulation,
causing
the
nerves
to
show
a
Wedensky
effect
when
fatigued
(Wedenskyl).
Therefore
for
the
later
experiments
an
apparatus
was
used
with
a
revolving
brush
contact
by
means
of
which
the
stimuli
could
be
varied
in
frequency
as
desired
from
6-40.
-In
practice
it
was
found
that
a
rate
of
14-16
per
sec.
gave
the
best
results,
that
is
to
say
tetanus
without
any
possibility
of
the
Wedensky
effect.
It
was
always
possible
to
tell
the
presence
or
absence
of
this
effect
by
the
fact
that
the
muscle
gave
a
preliminary
twitch
at
the
instant
of
turning
on
the
current,
in
the
manner
described
by
Adrian
and
Lucas.
Heat
production
of
the
current.
As
stated
above,
the
method
of
indirect
experiment
was
adopted
after
trying
the
direct,
because
the
amiount
of
current
required
to
excite
is
relatively
extremely
small.
The
heat
produced
by
the
current
was
determined
experimentally
under
the
conditions
of
the
experiments
by
passing
the
current
for
the
same
time
as
had
been
employed
throughout
the
experiment
4nd
observing
any
increased
rise
of
temperature
in
the
flask.
This
was
never
found
to
be
greater
than
from
1-2
scale
divisions
of
the
galvanometer.
Calibration
of
thermopile.
The
light
was
reflected
fromn
the
galvano-
iueter
on
to
a
fixed
scale
and
the
thermopile
calibrated
directly
(in
the
same
way
that
Hill
calibrated
his
apparatus).
That
is
to
say
a
difference
of
temperature
of
about
1°C.
was
made
between
the
flasks,
determined
carefully
with
an
accurate
Beckmann
thermometer;
the
galvanometer
deflection
was
then
compared
with
this.
The
average
of
several
determinations
gave
10
C.=
332
scale
divisions
(individual
determina-
tions
varied
between
334
and
330).
In
the
reading
of
the
deflection,
and
in
all
the
other
galvanometer
readings,
the
readings
were
the
differences
of
the
deflections
obtained
by
reversing
the
current
through
the
galvanometer.
For
instance
if
the
galvanometer
for
a
deflection
right
of
the
zero
read
400,
and
left
read
150,
the
reading
taken
would
be
400
-
150
=
250
scale
divisions.
Experimental
procedure.
In
all
the
experiments,.other
than
those
in
Table
I
2,
the
following
method
was
employed.
1
Wede
n
sk
y.
Arch.
f.
d.
ges.
Physiol.
xxxvii.
p.
69.
1885.
2
In
the
earlier
experiments
(Table
I)
an
apparatus
was
used,
which
was
only
appli.
cable
to
one
limb
pair,
therefore
the
method
was
much
more
liable
to
error.
The
results
are
given
for
comparison.
254
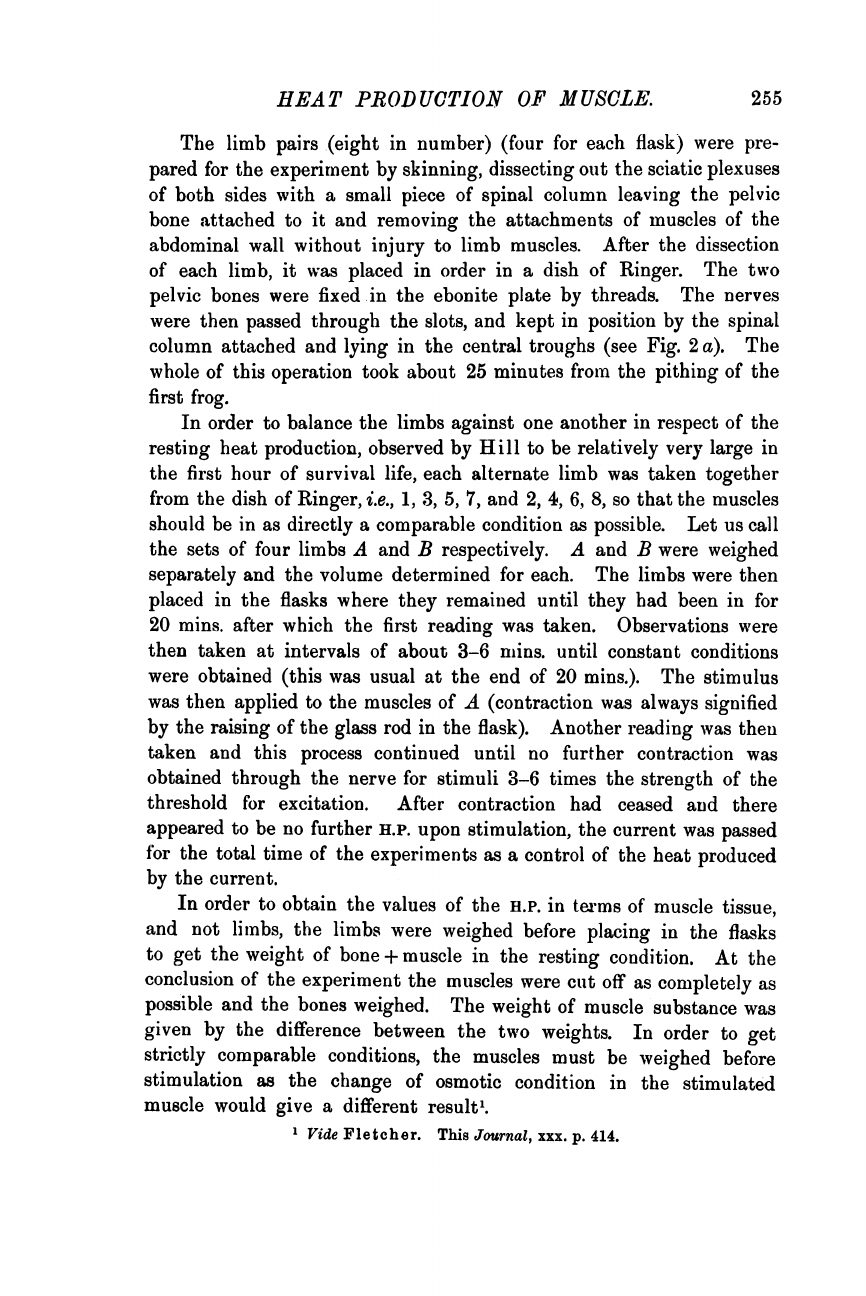
HEAT
PRODUCTION
OF
MUSCLE.
The
limb
pairs
(eight
in
number)
(four
for
each
flask)
were
pre-
pared
for
the
experiment
by
skinning,
dissecting
ouit
the
sciatic
plexuses
of
both
sides
with
a
small
piece
of
spinal
column
leaving
the
pelvic
bone
attached
to
it
and
removing
the
attachments
of
muscles
of
the
abdominal
wall
without
injury
to
limb
muscles.
After
the
dissection
of
each
limb,
it
was
placed
in
order
in
a
dish
of
Ringer.
The
two
pelvic
bones
were
fixed
in
the
ebonite
plate
by
threads.
The
nerves
were
then
passed
through
the
slots,
and
kept
in
position
by
the
spinal
column
attached
and
lying
in
the
central
troughs
(see
Fig.
2
a).
The
whole
of
this
operation
took
about
25
minutes
froin
the
pithing
of
the
first
frog.
In
order
to
balance
the
limbs
against
one
another
in
respect
of
the
resting
heat
production,
observed
by
Hill
to
be
relatively
very
large
in
the
first
hour
of
survival
life,
each
alternate
limb
was
taken
together
from
the
dish
of
Ringer,
i.e.,
1,
3, 5,
7,
and
2,
4,
6,
8,
so
that
the
muscles
should
be
in
as
directly
a
comparable
condition
as
possible.
Let
us
call
the
sets
of
four
limbs
A
and
B
respectively.
A
and
B
were
weighed
separately
and
the
volume
determined
for
each.
The
limbs
were
then
placed
in
the
flasks
where
they
remained
until
they
had
been
in
for
20
mins.
after
which
the
first
readino
was
taken.
Observations
were
then
taken
at
intervals
of
about
3-6
nmins.
until
constant
conditions
were
obtained
(this
was
usual
at
the
end
of
20
mins.).
The
stimulus
was
then
applied
to
the
muscles
of
A
(contraction
was
always
signified
by
the
raising
of
the
glass
rod
in
the
flask).
Another
reading
was
then
taken
and
this
process
continued
until
no
further
contraction
was
obtained
through
the
nerve
for
stimuli
3-6
times
the
strength
of
the
threshold
for
excitation.
After
contraction
had
ceased
and
there
appeared
to
be
no
further
H.P.
upon
stimulation,
the
current
was
passed
for
the
total
time
of
the
experiments
as
a
control
of
the
heat
produced
by
the
current.
In
order
to
obtain
the
values
of
the
H.P.
in
terms
of
muscle
tissue,
and
not
limnbs,
the
limbs
were
weighed
before
placing
in
the
flasks
to
get
the
weight
of
bone
+
muscle
in
the
resting
condition.
At
the
conclusion
of
the
experiment
the
muscles
were
cult
off
as
conmpletely
as
possible
and
the
bones
weighed.
The
weight
of
muscle
substance
was
given
by
the
difference
between
the
two
weights.
In
order
to
get
strictly
comparable
conditions,
the
muscles
must
be
weighed
before
stimulation
as
the
change
of
osmotic
condition
in
the
stimulated
muscle
would
give
a
different
result'.
I
Vide
Fletcher.
This
Journal,
xxx.
p.
414.
255

R.
A.
PETERS.
Calculation
of
actual
value
of
heat
production.
The
temperature
differences
between
the
two
flasks,
obtained
as
galvanometer
scale
divisions,
were
plotted
on
squared
paper
as
ordinates,
the
abscissae
representing
intervals
of
time.
They
were
corrected
for
heat
loss
by
the
method
described
by
Hill',
by
multiplying
the
ordinate
at
the
middle
of
a
given
period
by
the
coefficient
of
heat
loss
and
correcting
the
points
upon
the
observed
curve
accordingly.
It
has
been
shown
by
Hill
that
the
resting
heat
production
is
a
comparatively
large
quantity,
especially
in
the
first
few
hours.
In
any
calculation
of
heat
produced
during
stimulation
over
long
periods,
this
has
to
be
taken
into
account.
The
method
as
finally
modified
will
be
described.
Figs.
3
and
4
are
two
of
the
actual
curves
of
heat
production
obtained
and
chosen
at
random
from
the
experiments
given
in
Table
II.
The
upper
irregular
curves
are
the
observed
curves
of
heat
production.
Periods
of
stimula-
tion
referred
to
approxinmate
current
strengths
are
marked
in
black
patches.
Before
stimulating
observations
were
continued
for
periods
of
10-20
minutes
in
order
to
obtain
a
sufficiently
even
curve
for
continua-
tion
as
a
base
line.
The
lower
curve
is
drawn
through
the
points
of
correction.
The
resting
curve
corrected
for
heat
loss
is
continued
as
three
black
lines,
of
which
the
middle
is
drawn
through
the
corrected
points,
the
other
two
lines
representing
the
approximate
limits
of
the
experimental
error
involved
in
the
continuation
of
the
base
line.-
As
the
galvanometer
cannot
be
read
accurately
to
less
than
*5
of
a
scale
division,
the
experimental
error
of
any
observation
may
be
one
division
npon
either
side
of
the
observed
point:
this
is
indicated
in
the
diagram
by
a
small
rectangle.
The
error
in
time
cannot
be
more
than
*5
of
a
minute.
When
stimulation
ceases
to
cause
a
further
heat
evolution,
the
number
of
scale
divisions
between
the
position
of
the
corrected
curve
for
stimulation
and
the
continuation
of
the
line
for
the
resting
heat
production
is
reckoned
as
the
heat
evolved
in
contraction
of
the
muscle
to
a
state
of
fatigue.
A
description
of
further
points
will
be
given
under
the
experimental
results.
Results
of
experiments.
In
the
earliest
experiments,
to
be
quoted
in
Table
I,
one
frog's
limb
was
used
in
each
flask,
that
in
the
control
flask
being
dead.
The
resting
heat
production
was
therefore
very
large,
and
observations
had
to
be
continued
for
a
very
long
time,
30
minutes
or
more,
in
order
to
get
even
approximate
accuracy
in
continuing
a
line
drawn
through
them.
The
I
This
Journal,
XLIII.
p.
274.
256

HEAT
PRODUCTION
OF
MUSCLE.
TABLE
I.
Heat
production
in
muscles,
dead
used
as
controls.
muscles
being
Calories
(gram)
per
c.c.
TemPeratur
limb
skinned
C.
A
1
041
13
A
2
063
14
A
3
050
17
A
4
022
16
A
5
0-42
15
A
6
042
15
A
7
0-23
14
A
8
020
11
A
9
0
49
15
A
10
0.50
17
A
11
070
19
A
12
044
19
A
13
0-31
14-5
Average
0-42
oals.
per
c.
e.
Calories
(gram)
e
per
gm.
muscle
(weight)
1P08
Weighed
after
0-76
L
CHCl3
rigor,
0-36
I
therefore
0.57)
values
high.
1.
)
Weight
after
0
60
J
stimulation
Average
*78
Rate
of
stimulation
100-200
per
seC.
25
per
seC.
30
I,
14
,,
13
it
50
,,
errors
involved
in
this
method
of
control
by
a
dead
muscle
were
magnified
by
the
steepness
of
the
line
of
resting
heat
production,
and
are
in
all
probability
extremely
large.
It
is
considered
to
be
worth
while
to
record
the
results,
as
the
average
is
certainly
close
to
that
obtained
by
the
more
accurate
methods
used
for
Table
II.
The
results
are
seen
to
be
extremely
variable,
the
wide
variations
may
be
due
either
to
the
conditions
of
the
frogs
(they
were
used
at
the
breeding
season)
or
to
difficuilties
of
observation.
As
far
as
they
can
be
trusted,
they
seem
to
indicate
a
fact
proved
later
that
heat
produced
during
periods
of
stimulation
leading
to
fatigue
is
approximately
half
that
produced
in
chloroform
rigor
upon
the
fresh
muscle.
For
this,
Hill
found
about
10
cal.
per
c.c.,
of
which
the
value
42
cal.
appeared
to
be
nearly
one
half.
This
point
however
came
out
much
more
clearly
in
the
later
experiments.
In
the
experiments
described
in
Table
II,
of
which
examples
are
given
in
Figs.
3
and
4,
the
difficulty
connected
with
the
resting
heat
production
was
minimised
and
practically
removed
in
most
cases,
by
using
muscles
in
the
control
flask
which
had
been
treated
in
exactly
the
same
manner
as
those
prepared
for
stimulation.
In
this
manner,
it
was
possible
approximately
to
balance
the
resting
heat
productions
differentially
against
one
another.
Although
it
was
practically
never
possible
to
obtain
exactly
similar
pairs
of
limbs,
the
value
of
the
method
of
control
is
certain,
because
of
the
near
approach
of
the
line
for
the
resting
heat
production
to
the
horizontal.
257
r4

258
R.
A.
PETERS.
Figs.
3
and
4
representing
Exps.
3
and
6,
of
which
the
full
details
are
given
below,
illustrate
the
two
extremes
of
balance
obtained
in
the
experiments
of
Table
II.
Fig.
3
is
one
of
the
best
conditions
of
balance;
Fig.
4
the
worst.
It
is
seen
that
in
Fig.
4,
the
balance
is
quite
close,
being
in
fact
closer
than
any
of
the
curves
of
Table
I.
That
the
Scale
ivisio
Ext
emp.
Curve
-
1
90
100
110
120
I3S
140
160
Fatigue
loot~
170O
180
X
__
-0~~~~~~~~~~-
~~~~~0
--
.6
I
I
I
f
.
I
/
..-
.
I
.'
,
;1
Dio
I2'ZO
I5
jtS0i.
1901
200
210
220
-32n
-
I
90
1
100
1110
I
1U0
1
1301
1140
160
f
1
o
170)
Minutes
*0
1
.2
3
4
*5
6
7
a
s
10
1
I
Hours
Fig.
3.
Curve
of
heat
evolution
for
stimulation
to
fatigue
in
Exp.
3,
Table
I.
Ordinates=scale
divisions
of
galvanometer.
Abseissw=minutes
from
pithing,
and
decimal
points
of
one
hour
from
beginning
of
observation.
Black
rectangles
represent
times
of
stimulation
with
the
approximate
current
strengths
represented
as
ordinates.
Upper
curve=
observed
curve
of
heat
evolution.
Lower
curve
(dotted)=curve
corrected
for
heat
loss.
The
part
of
curve
before
stimulation
is
conitinued
as
a
dark
line,
being
the
curve
for
resting
heat
production.
The
rectangles
round
two
observed
points
represent
approximate
experimental
error
of
reading,
the
two
lines
on
either
side
being
drawn
to
obtain
the
experimental
error
of
the
observations
of
heat
production.
The
bracket
shows
the
number
of
scale
divisions
produced
by
stimulation.
(External
temperature
curve
shows
variations
in
temperature
conditions
surrounding
flasks
during
period
of
experiment.)
divergence
of
the
line
from
the
horizontal
in
Fig.
4
is
not
due
to
a
bad
correction
for
heat
loss,
is
certain
from
the
fact
that
the
weight
of
the
muscles
used
in
the
control
flask
was
3
grams
heavier
in
this
case,
so
that
they
would
be
expected
to
give
a
greater
heat
production
and
cause
the
direction
of
the
corrected
line
found
in
Fig.
3.
0
IG
r
.DIvIsions
ZfO
-129
go
S.Oiv.-

HEAAT
PRODUCTION
OF
MUSCLE.
259
In
all
the
experiments
30
c.c.
of
Ringer
were
placed
in
each
flask.
The
small
differences
caused
by
differences
in
volume
amount
of
solution
in
the
flasks
were
not
large
enough
to
make
appreciable
errors.
Details
of
one
experiment
(Exp.
4)
are
given,
the
others
were
performed
in
a
similar
manner.
Fig.
4.
Fatigue
Exp.
6.
For
description
refer
to
Fig.
3.
The
last
stimulation
period
of
6
mins.,
3
mins.
at
current
strength
4,
and
3
mins.
at
8,
represents
control
of
heat
produced
by
current.
TABLE
II.
Heat
production
in
muscles
(April-May),
living
muscles
being
used
as
controls.
1
2
3
4
5
6
7
8
9
10
11
Stim.
Time
H.
P.
Linmb
Muscle
Limb
rate
of
stim.,
No.
of
Heat*
Ext.
temp.
weight,
weight,
vol.,
per
stim.,
in
limbs
per
gm.
Temp.
varied
Exp.
gms.
gms.
c.c.
sec.
mins.
Sc.
Div.
used
musc.
IC.
between
1
33-65
.
18-8
31
17
7
81
4
*93
16
9
169-17s5
2
31-0
18-8
28
38
7
.55
4
-61
18
5
18-3-18-5
3
35-4
22-2
33-5
16
9
91
5
*91
18-7
18
8-19
2
4
33.4
21-2
31
8
8
67
4
*68
19
2
1910
5
27-07
16-5
25
11
5
74
4
*90
17-4
16-8-17
2
6
33
05
20-5
30
14
8
90
4
-94
14
14-14-4
7
27-1
17-0
25
16
8
81
4
*92
17-8
17-18
8
27
7
16'6
(26)t
13
8
55-5
4
'67
14
5
14-1-14
7
*
Heat
production
throughout
this
paper
is
measured
in
gram
calories.
t
Volume
arrived
at
by
averaging
the
others.

260
R.
A.
PETERS.
Exp.
3.
(Fig.
3.)
Limbs
of
frogs
1,
3,
5,
7
(A)
used
for
stimulation
flask.
Limbs
of
frogs
2,
4,
6,
8
(B)
for
control.
Total
weight
of
(A)
=
3540
gms.
Weight
of
bones
only
=
13-20
,
Weight
of
muscle
=
22
20
,,
Volume
33-5
c.c.
Total
weight
of
(B)
=
33A40
gms.
Weight
of
bones
only
=
12
90
,
Weight
of
muscle
=
20
50
9,
Volume
31
a.c.
Time
represents
minutes
from
pithing
of
the
first
frog.
Dissection
took
20
minutes.
Time
Galvanometer
X
96
220
99
220
102
217
107
216
110
211
113
209
116
208
119
208
Stimulation
for
4
minutes.
Current
strength
3.
Rate
100
revolutions
in
50
secs.
=800
stimulations
in
50
secs.
-16
per
sec.
125
144
128
136f5
reading
Time
(contd.)
Galvanometer
reading
131
131-5
134
128-5
Stimulation
for
2
minutes
at
same.
138
115-6
140
113'5
147
109
Stimulation
for
2
minutes.
154
107
156
102-5
159
102-5
Stimulation
for
1
minute.
162
101.5
Control
of
heat
production
not
done
in
this
experiment.
Correctionfor
heat
loss.
Constant
k=
160.
Divisions
of
an
hour
represented
on
the
curve
for
convenience
as
decimal
points.
(Fig.
3.)
Period
of
an
hour
...
0-*1
*1--3
.3-.4
4-'6
*6-*7
*7-'8
*8*9
-9-1
0
1*0-1
1
Value
of
ordinate
m
at
219
214
208
141
125
112-5
109
107-5
102-5
middle
of
period
(m
x
k)
...
...
3-51
6.85*
3.33
4.52*
2'0
1-80
1P75
1-72
1-64
H
fmx
k+total
heat}
3'51
10
36
13-69
18-21
20-21
22'01
23'76
25,48
27-12
Value
of
ordinate
at
217
209
207
130-5
115
111
108
105
101.5
end
of
period
(e)
e+H
...
...
220&5
219-4
220-7
148-7
135-2
133-0
131-8
130-5
128-6
*=2mx
k.
Difference
between
curves
of
stimulation
and
resting
heat
production
=
91
scale
divisions.
Approximate
limits
of
error
due
to
projection
=88-94
scale
divisions.
This
error
represents
the
divergence
in
the
two
thinner
lines
on
either
side
of
the
line
of
resting
heat
production,
being
the
projection
of
the
small
galvanometer
reading
error
of
2
%/0
approximately.
Calculation
of
heat
production'.
10
C.
=
332
scale
divisions.
Muscle
weight=35,4
gms.,
specific
heat=0'83,
water
equi-
valent
of
limbs=29-4
c.c.
1
Throughout
this
paper
the
specific
heat
of
the
tissue
is
taken
to
be
0-83,
the
approxi-
mate
value
given
by
Pembrey,
Schafer's
Text-Book
of
Physiol.
i.
p.
839.

HEAT
PRODUCTION
OF
MUSCLE.
Temperature
of
flask
and
contents
has
been
raised
91
OC.
Flask
contains
muscle
332
(29.4
c.c.)
+Ringer
(vol.
30
c.c.)
+heat
capacity
(14
c.c.)
=294
+
30
+
14=734.
Hence
22-2
gms.
of
muscle
have
evolved
heat
sufficient
to
raise
73-4
c.c.
water
09
0
C.
332
Or,
heat
production
per
gm.
of
muscle
during
stimulation
to
fatigue
91x
c32
calories=
-91
calorie.
~32'22-20
-
Approximate
limits
of
error
due
to
projection of
curve
of
resting
heat
production
=
88-94
calories.
The
result
given
in
column
nine
vary
from
i62-'94
calories
per
gram
of
muscle
tissue,
falling
into
two
groups.
This
might
seem
to
indicate
the
existence
of
two
processes.
But
if
two
processes
did
exist,
it
is
feared
that
the
method
may
not
be
sufficiently
accurate
to
decide
between
them,
owing
to
the
experimental
error
to
which
these
results
must
be
liable.
The
absolute
experimental
error
is
difficult
to
estimate
as
it
depends
upon
(1)
an
error
concerned
with
the
projection
of
the
small
error
of
reading
the
galvanometer
(about
2e/o)
in
the
continua-
tion
of
the
line
of
resting
heat
production,
amounting
on
the
average
to
10
0/0
of
the
total
reading;
(2)
an
error
introduced
by
the
inadvertent
cutting
of
small
nerve
branchesi,
and
damaging
of
nerves
in
some
cases
at
their
attachuient
to
the
vertebral
column
in
order
to
get
the
piece
of
bone
sufficiently
small
to
fit
into
the
stimulation
trough
(Fig.
2b).
This
would
produce
a
low
value
owing
to
the
inclusion
of
unfatigued
muscles
in
the
calculation;
(3)
small
errors
in
heat
gain
or
heat
loss
in
the
calorimeter.
Therefore
taking
into
account
errors
(1)
and
(3)
which
might
be
in
either
direction,
and
the
error
(2)
which
must
always
be
a
low
error,
it
is
possible
that
the
low
results
between
-6-17
are
to
be
attributed
to
error
(2)2.
As
the
object
was
to
discover
the
maximum
heat
production
of
stimulation
to
fatigue,
the
average
of
93,
*91,
-90,
94,
-92
=
92
calorie
will
be
taken
as
representing
the
highest
value
obtainable
in
such
periods
of
stimulation.
It
will
be
remembered
that
the
values
for
heat
production
of
chloroform
rigor
obtained
by
Hill
varied
from
*7-1-2
cals.
per
c.c.
of
frog's
limbs,
from
which
it
certainly
seemed
the
case
that
H.P.
of
1
In
getting
out
the
preparation,
minute
dissection
was
sacrificed
to
speed
of
manipu-
lation.
2
The
experiments
below
in
Table
IV
further
make
it
probable
that
the
low
values
represent
want
of
fatigue
of
the
muscles
used.
If
two
tone
processes
existed,
it
would
be
expected
that
the
heat
production
of
chloroform
rigor
after
fatigue,
obtained
as
it
is
by
a
more
accurate
method,
would
also
show
such
division
of
results.
PH.
XLVII.
17
261

R.
A.
PETERS.
stimulation
was
not
more
than
of
the
order
of half
of
the
total
H.P.
of
rigor.
In
terms
of
c.c.
the
above
value
would
reduce
to
about
*6.
The
next
step
then,
was
to
determine
the
H.P.
of
CHC13
rigor
in
terms
of
grams
of
muscle
substance.
This
was
done
upon
a
large
scale
in
two
flasks
(kindly
lent
me
for
the
ptrpose
by
Mr
Hill)
which
were
exactly
balanced
as
regards
heat
loss
and
in
which
owing
to
their
size
the
coefficient
of
heat
loss
was
extremely
small.
The
limbs
ten
in
number
after
a
previous
weighing
were
made
up
to
the
required
volume
(300
c.c.)
with
neutral
Ringer,
the
whole
operation
being
performed
as
quickly
as
possible.
The
limbs
were
removed
without
dissecting
the
nerves
and
the
experiment
was
performed
as
described
in
Hill's
paper
with
the
exception
that
dead
muscles
were
placed
in
the
control
flask.
For
stirring,
the
muscles
lay
upon
a
plate
of
ebonite
to
which
was
attached
a
glass
rod
passing
through
a
hole
in
the
cork
of
flask.
To
avoid
any
possible
error
due
to
heat
of
friction,
both
flasks
were
stirred
equally.
Fig.
5
gives
the
curve
of
heat
evolution
obtained
in
Exp.
9.
The
heat
loss
was
1
scale
division
in
this
experiment.
The
chloroform
was
inserted
at
the
point
marked
by
an
arrow,
and
after
rapid
stirring,
the
first
reading
taken.
By
a
continuation
of
the
curve
backwards
until
it
becomes
parallel
to
the
ordinate,
the
beginning
of
heat
evolu-
tion,
which
takes
place
extremely
rapidly,
is
taken
as
occurring
at
20,
the
position
being
marked
by
a
cross.
The
observed
points
are
seen
to
fall
extremely
closely
upon
a
curve.
Heat
evolution
in
scale
divisions
extends
from
-
20
-.
+
68,
=88
scale
divisions,
+1
scale
division
heat
loss
=
89
scale
divisions.
Heat
capacity
of
flask=20
c.c.
10
C.
=327
scale
divisions.
Total
volume
of
water
+
limbs
+
chloroform
10
c.c.
=
300
c.c.
Water
equivalent
of
limbs=67-7.
Volume
of
limbs
=92
c.c.
Therefore
heat
production
per
gram
of
muscle
substance
89
295-7
=2_7
x
4794
=
1694
cals.
The
results
obtained
corrected
for
heat
loss,
where
necessary,
were
as
follows:
TABLE
HI.
Number
of
Limbs
Muscle
H.
P.
in
Heat
Temp.
Number
of
experiment
weight
weight
scalediv.
per
gm.
C.
imb
pairsused
9
80
64
47-46
84
1-69
15
10
10
98-20
58-36
105
1-70
1385
10
11
7718.
44588
80
1,70
13-5
10
262

HEAT
PRODUCTION
OF
MUSCLE.
The
method
of
finding
the
heat
production
of
chloroform
rigor
admits
of
an
accuracy
considerably
greater
than
that
of
experiments
in
Table
II,
owing
to
the
fact
that
the
experiment
is
done
upon
a
larger
number
of
limbs,
in
a
larger
flask
with
a
much
smaller
coefficient
of
heat
loss;
it
is
seen
that
the
results
for
maximum
heat
production
are
remarkably
constant
and
seem
to
have
eliminated
any
error
due
to
possible
variability
of
tissue
utilised.
Fig.
5.
Exp.
9,
Table
III.
Heat
evolution
of
chloroform
rigor.
Ordinates=scale
divisions.
Abscissie=minutes.
Arrow
represents
introduction
of
chloroform,
and
first
reading.
The
two
crosses
mark
beginning
and
end
of
heat
evolution.
The
curve
passes
through
the
galvanometer
zero,
making
calculation
for
heat
loss
unnecessary.
They
average
1-70
cals.
per
gram.
The
close
agreement
of
these
three
figures
is
probably
fortuitous.
Thus
the
heat
production
of
stimulation
to
fatigue
bears
to
the
heat
production
of
chloroform
rigor
in
the
fresh
muscle
roughly
the
same
proportion
as
observed
by
Fletcher
and
Hopkins
for
lactic
acid
production,
ie.
approximately
one
half.
It
was
shown
by
Hill
that
stimulation
reduced
the
H.P.
of
CHCI8
rigor
very
markedly.
There
were
therefore
grounds
for
thinking
that
the
sum
of
the
H.P.
of
stimulation
and
H.P.
of
rigor
after
stimulation
17-2
263

R.
A.
PETERS.
was
not
more
than
the
total
H.P.
of
CHC1,3
rigor
in
the
fresh
muscle.
Experiments
were
done
to
determine
the
heat
production
of
CHCI3
rigor
after
prolonged
stimulation.
In
all
the
experiments,
given
in
Table
IV,
the
limbs
were
stimulated
as
in
the
preceding
experiments,
for
5-10
minutes,
at
rate
of
14-16
per
sec.
TABLE
IV.
Heat
production
of
chlorofornm
rigor
for
fatigued
muscles.
Number
Limb
Muscle
H.
P.
Heat
pr.
Temp.
Exp.
No.
of
limbs
weight
weight
(scale
dive.)
pergm
gC.
12
10
98-25
71-76
40
*86
18'5
13
10
78-3
31'7
33
.99
19
*14
11
81-52
51.01
75
.91*
17'5
15
9
49-20
28-93
37
*82
18
16
10
86-62
53-81
47
*80
17-5
*17
9
95'96
60-88
70
1.04*
17-5
In
each
of
the
experiments
marked
with
an
asterisk,
it
was
observed
that
one
of
the
large
nerves
going
to
one
limb
of
one
of
the
frogs
was
inadvertently
eut.
The
result
is
on
the
high
side,
which
is
in
accordance
with
expectation.
The
gross
variation
of
these
values
is
-80-1-04
calories
per
gram.
The
possible
error
is
greater
than
in
Table
III,
as
there
is
only
half
the
heat
evolution,
and
less
than
in
Table
II,
as
the
error
(1)
mentioned
after
Table
II
above
does
not
occur.
Cutting
nerves
would
have
the
effect
of
giving
an
error
upon
the
high
side,
which
was
observed
in
experiments
14
and
17.
Exp.
13
was
an
earlier
one,
in
which
no
special
note
was
taken
of
possible
nerve
cutting.
An
average
of
the
values
in
Table
IV
excluding
experiments
14
and
17,
which
are
known
to
be
invalid
owing
to
nerve
cutting,
is
-87
calorie
per
gram
of
muscle.
From
this
it
follows
that
the
diminution
of
the
value
of
chloroform
rigor
for
the
unstimulated
muscle
(Table
III),
if
there
be
no
other
process
involved
in
fatigue
than
in
rigor,
should
be
1-70--87
calories
=
'83
calorie.
From
Table
II,
the
heat
production
of
fatigue
was
shown
to
have
a
maximum
value
of
*92
calorie
per
gram,
a
value
sufficiently
close
to
*83
calorie
per
gram
to
make
it
probable
that
the
diminution
observed
in
Table
IV
is
a
diminution
of
the
heat
producing
process
of
Table
III.
With
regard
to
the
lower
values
*61,
*68,
'67
calories
in
Table
II,
which
it
was
considered
could
be
explained
by
want
of
stimulation,
in
experiment
17,
where
a
nerve
was
cut,
1X04
calorie
was
obtained.
1'70-1-04
calories
would
be
*66
calorie
per
gram.
It
is
upon
this
ground
that
I
feel
that
the
low
values
are
experimental.
264

HEAT
PRODUCTION
OF
MUSCLE.
If
this
be
the
case,
the
answer
to
the
second
question
is
that
the
maximum
heat
production
of
contraction
plus
the
heat
production
of
chloroform
rigor
in
a
similarly
fatigued
muscle
is
roughly
equal
to
the
heat
production
of
chloroform
rigor
in
the
fresh
muscle.
For
from
Table
II,
we
have
for
the
maximum
heat
production
of
contraction
*92
calorie
per
gram
as
an
average,
from
Table
IV
for
the
heat
production
of
chloroform
rigor
87
calorie
per
gram
as
an
average,
of
which
the
sum
1-79
calories
is
within
experimental
error
of
1'70
calories,
the
value
from
Table
III
for
the
chloroform
rigor
of
the
fresh
muscle.
So
it
wouild
seem
that
any
other
change
beyond
that
occurring
simultaneously
with
lactic
acid
liberation
must
involve
only
small
heat
changes'.
In
other
words
it
would
appear
from
these
results
that
the
processes
of
contraction
and
those
of
rigor
do
make
calls
upon
the
same
precursor.
The
next
question
was,
whether
the
presence
of
a
large
amount
of
oxygen
would
upset
the
relation
established
above.
The
experiments
were
made
in
two
ways:
In
one
the
maximum
heat
production
of
fatigue
was
estimated
and
the
muscle
transferred
to
a
high
pressure
of
oxygen2
for
a
time
and
then
a
further
estimation
made.
Slight
evidence
of
a
further
heat
evolution
of
about
*2
calorie
was
obtained
in
a
few
experiments,
but
the
method
of
indirect
stimulation
was
not
found
applicable
owing
to
the
difficulty
of
keeping
up
the
effect
of
the
nerves
in
the
muscle.
In
the
other
method,
the
muscles
were
placed
in
the
Ringer's
fluid
previously
saturated
with
oxygen,
under
pressure,
and
oxygen
was
bubbled
through
the
fluid
for
a
time,-depending
upon
the
continuance
of
the
effect
of
the
nerve
in
the
muscle,
the
maximum
being
1I
hours,-
the
muscles
were
then
removed
and
the
heat
production
estimated
as
quickly
as
possible.
It
was
not
possible
to
reduce
the
period
from
the
time
of
removal
of
the
muscle
from
the
02
pressure
to
the
time
of
observing
stimulation
to
less
than
20
minutes,
10
minutes
for
fixing,
and
10
minutes
for
observing
curve;
and
sometimes
it
took
longer
to
attain
settled
con-
ditions
in
temperature
of
the
flasks.
The
results
of
these
experiments
were:
A
method
of
avoiding
this
contingency
by
estimating
the
heat
production
of
chloro.
form
rigor
after
fatigue
upon
the
muscles
in
the
actual
flask
used
for
determining
the
heat
production
of
stimulation
I
tried
at
first,
but
was
obliged
to
abandon
as
the
errors
involved
in
measuring
the
slow
heat
production
of
chloroform
rigor
in
the
apparatus
designed
for
measuring
the
heat
production
were
too
great.
2
The
pressure
of
oxygen
used
was
one
atmosphere.
265

R.
A.
PETERS.
TABLE
V.
Time
Stim.
H.
P.
Stim.
of
rate
of
No.
Heat
lasted,
Exp.
oxygen,
Limb
Muscle Muscle
per
stim.
of
per
Temp.
Ext.
temp.
mins.
No.
mins.
weight
weight
vol.
sec.
in
S.D.
limb
gm.
cals.
IC.
*C.
8
18
90
27'29
17'2
27
16
76
4
'88
14
13'8-14'0
10
19
50
30'67
18'1
29
20
40
4
*67
1388
13'0-14'0
7
20
45
29'38
19'0
27-5
11
70'5
4
'76
14'3
1388-14'3
6
21
60
27'19
16'25
24-5
16
76
4
-94
15'8
16'0
It
will
be
seen
that
the
heat
production
per
gram
of
muscle
varied
from
'70-'94
calories,
which
is
not
greater
than
the
value
obtained
for
muscles
which
had
not
been
treated
witb
oxygen.
The
experiments
indicate
that
the
muscle
cannot
store
oxygen
but
are
not
decisive,
since
the
pressure
may
not
have
been
sufficiently
high
to
drive
the
oxygen
into
the
muscle
substance,
or
the
time
necessarily
elapsing
between
the
estimation
of
the
heat
production
and
the
removal
from
the
pressure
bottle,
may
have
allowed
the
escape
of
any
oxygen
that
had
penetrated
the
muscle
substance.
So
far
as
the
experiments
can
be
trusted,
however,
they
seem
to
indicate
that
the
muscle
cannot
store
oxygen
as
such,
a
conclusion
which
has
been
reached
by
other
observers.
An
experiment
of
the
nature
of
those
in
this
paper,
but
employing
direct
stimulation,
should
be
able
to
settle
the
question
definitely.
Production
of
lactic
acid.
As
was
stated
above,
it
was
shown
by
Hill
that
there
was
a
close
relation
between
the
production
of
lactic
acid
and
the
liberation
of
heat.
Now
it
was
shown
by
Fletcher
and
Hopkins
that
there
was
a
maximum
production
of
lactic
acid
varying
in
different
months
between
about
'35-'55
0/
(measured
as
Zn
lactate)
in
summer,
'4
e/o
approximately.
When
they
stimulated
to
fatigue
over
varying
periods
of
time
and
for
different
strengths
of
current,
they
obtained
values
for
fatigue
of
about
half
those
obtained
in
total
rigor.
To
quote
their
words,
"The
average
percentage
yield
is
'216
O/0,
of
which
the
highest
yield
was
'28
%h,
the
lowest
'147
O/o,
and
13
of
the
determina-
tions
lay
between
'18-'25
0h.
One
experiment
in
which
stimulation
through
the
sciatic
plexuses
was
employed,
gave
a
yield
of
'203
0/f.
In
the
introduction
to
this
paper,
it
will
be
remembered
that
one
of
the
objects
in
view
was
to
establish
further
the
fact
that
any
process
tending
to
liberate
lactic
acid
in
a
muscle
tended
to
liberate
heat,
which
bas
been
confirmed
by
the
values
for
heat
production
obtained.
Still
further
another
point
would
seem
to
be
established,
namely,
that
in
contraction
heat
production
and
lactic
acid
liberation
are
extremely
266
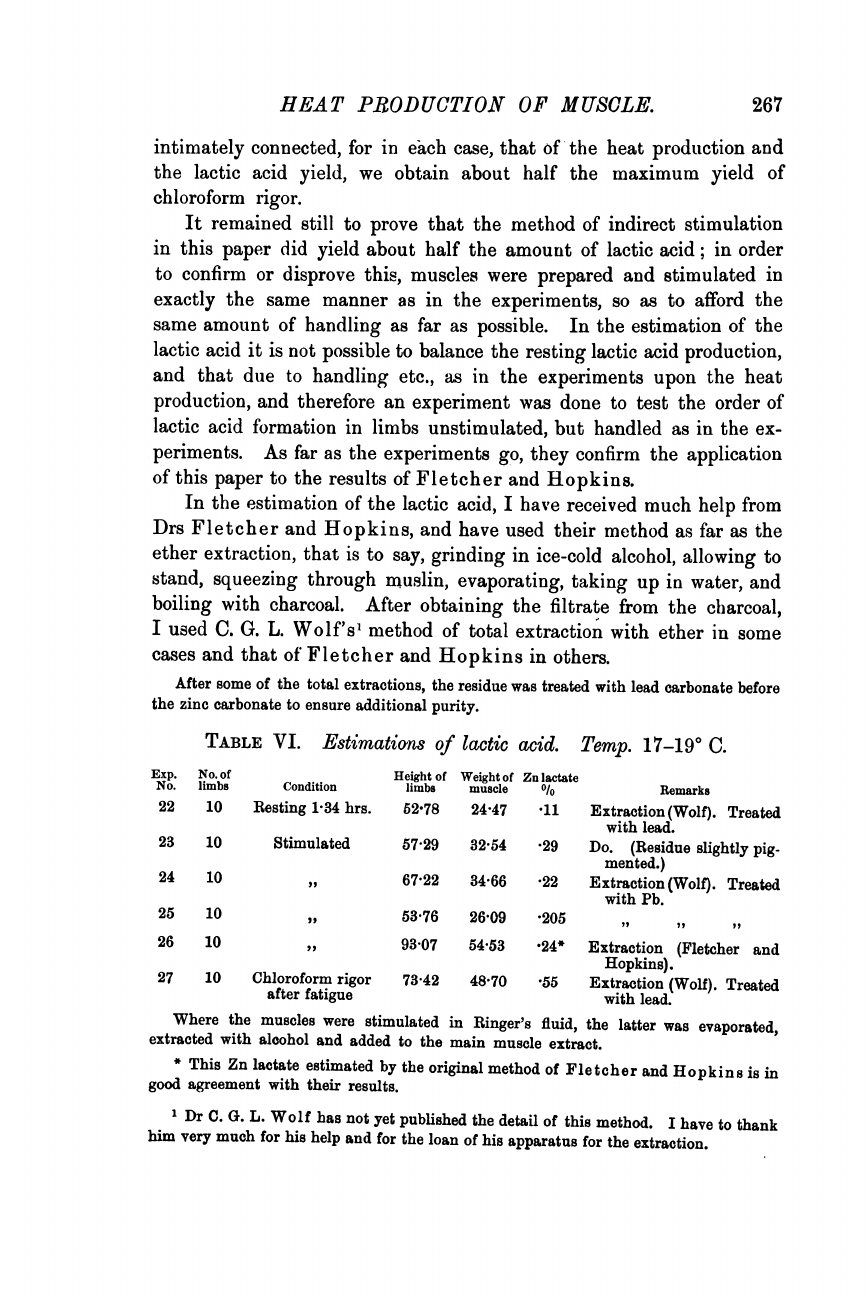
HEAT
PRODUCTION
OF
MUSCLE.
intimately
connected,
for
in
each
case,
that
of
the
heat
prodtuction
and
the
lactic
acid
yield,
we
obtain
about
half
the
maximum
yield
of
chloroform
rigor.
It
remained
still
to
prove
that
the
method
of
indirect
stimulation
in
this
paper
did
yield
about
half
the
amount
of
lactic
acid;
in
order
to
confirm
or
disprove
this,
muscles
were
prepared
and
stimulated
in
exactly
the
same
manner
as
in
the
experiments,
so
as
to
afford
the
same
amount
of
handling
as
far
as
possible.
In
the
estimation
of
the
lactic
acid
it
is
not
possible
to
balance
the
resting
lactic
acid
production,
and
that
due
to
handling
etc.,
as
in
the
experiments
upon
the
heat
production,
and
therefore
an
experiment
was
done
to
test
the
order
of
lactic
acid
formation
in
limbs
unstimulated,
but
handled
as
in
the
ex-
periments.
As
far
as
the
experiments
go,
they
confirm
the
application
of
this
paper
to
the
results
of
Fletcher
and
Hopkins.
In
the
estimation
of
the
lactic
acid,
I
have
received
much
help
from
Drs
Fletcher
and
Hopkins,
and
have
used
their
method
as
far
as
the
ether
extraction,
that
is
to
say,
grinding
in
ice-cold
alcohol,
allowing
to
stand,
squeezing
through
mnuslin,
evaporating,
taking
up
in
water,
and
boiling
with
charcoal.
After
obtaining
the
filtrate
from
the
charcoal,
I
used
C.
G.
L.
Wolf's'
method
of
total
extraction
with
ether
in
some
cases
and
that
of
Fletcher
and
Hopkins
in
others.
After
some
of
the
total
extractions,
the
residue
was
treated
with
lead
carbonate
before
the
zinc
carbonate
to
ensure
additional
purity.
TABLE
VI.
Estimations
of
lactic
acid.
Temp.
17-19o
C.
Exp.
No.
of
Height
of
Weight
of
Zn
lactate
No.
limbs
Condition
limbs
muscle
0/0
Remarks
22
10
Resting
134
hrs.
52-78
24-47
*11
Extraction
(Wolf).
Treated
with
lead.
23
10
Stimulated
57-29
32-54
*29
Do.
(Residue
slightly
pig-
mented.)
24
10
,,
67-22
34-66
*22
Extraction
(Wolf).
Treated
with
Pb.
25
10
,,
53-76
26-09
*205
,
..
26
10
,,
93
07
54.53
.24*
Extraction
(Fletcher
and
Hopkins).
27
10
Chloroform
rigor
73x42
48-70
*55
Extraction
(Wolf).
Treated
after
fatigue
with
lead.
Where
the
muscles
were
stimulated
in
Ringer's
fluid,
the
latter
was
evaporated,
extracted
with
alcohol
and
added
to
the
main
muscle
extract.
*
This
Zn
lactate
estimated
by
the
original
method
of
Fletcher
and
Hopkins
is
in
good
agreement
with
their
results.
I
Dr
C.
G.
L.
Wolf
has
not
yet
published
the
detail
of
this
method.
I
have
to
thank
him
very
much
for
his
help
and
for
the
loan
of
his
apparatus
for
the
extraction.
267

R.
A.
PETERS.
In
all
cases
where
stimulation
was
employed,
rate
was
14-16
per
sec.
and
stimulation
at
intervals
for
a
total
time
of
5-10
minutes.
The
results
are
of
the
samiie
order
as
those
of
Fletcher
and
Hopkins,
but
slightly
on
the
high
side.
This
is
what
would
be
expected,
taking
into
account
the
total
ether
extraction
employed
in
most
cases,
and
in
the
case
of
the
stimulated
muscles,
the
necessary
handling
in
dissecting
out
the
preparations.
In
Exp.
22,
there
is
an
attempt
to
form
some
idea
of
the
amount
of
acid
that
may
be
present
in
muscles,
which
have
been
treated
exactly
as
in
Exps.
23,
24,
25,
and
26,
with
the
exception
that
they
have
not
been
stimulated.
The
yield
of
acid
is
seen
to
be
rather
high,
especially
when
compared
with
the
-resting
minimum
of
Fletcher
and
Hopkins
of
.02O/o,
but
it
must
be
remembered
that
the
latter
was
obtained
by
placing
the
frogs
after
pithing
upon
ice
and
cutting
off
the
limbs
while
cold,
whereas
in
the
experiments
in
this
paper
the
muscles
were
kept
in
a
physiological
condition
for
subsequent
stimulation.
Discussion
of
Results.
It
has
been
seen
that
the
maximum
heat
production
of
contraction
to
a
condition
of
fatigue
in
an
excised
frog's
muscle
is
not
(as
might
be
perhaps
expected)
considerably
larger
than
that
of
clhloroform
rigor
in
the
fresh
muscle,
but
is
in
fact
about
one
half
of
its
value;
and
further
the
heat
production
of
the
chloroform
rigor
in
the
fatigued
muscle
will
account
for
the
other
half
of
the
heat
production,
so
that
contraction-
seems
to
make
calls
upon
the
same
process
as
does
the
condition
of
rigor.
The
ratio
of
the
heat
production
of
fatigue
to
that
of
chloroform
rigor,-one
of
approximately
one
half,-would
seem
to
have
an
interest-
ing
connection
with
the
experiments
of
Fletcher
and
Hopkins
upon
lactic
acid,
in
which
they
found
a
ratio
of
the
same
order.
The
relative
constancy
of
the
ratio
would
seem
to
merit
further
investigation,
as
it
is
possible
it
is
dependent
upon
some
property
of
the
muscle.
Also
I
have
observed
in
these
experiments
a
point
which
Dr
Fletcher
has
told
me
that
they
observed
in
their
experiments
upon
lactic
acid
production,
namely
that
upon
reaching
a
certain
point
of
fatigue-a
point
which
was
reached
extremely
quickly-further
stimulation
seemed
to
be
without
much
influence
upon
the
heat
production.
At
first,
I
noted
most
carefully
that
the
first
periods
of
stimulation
and
second
etc.
should
agree
in
duplicate
experiments,
but
later
I
found
that
provided
the
stimulation
had
taken
4-6
mins.
altogether,
the
greatest
268

HEAT
PRODUCTION
OF
MUSCLE.
part
of
the
heat
production
had
always
appeared.
This
maximum
appearance
too
seemed
in
many
cases
to
be
before
the
complete
cessation
of
irritability;
a
suggestion
of
the
same
fact,
Dr
Fletcher
has
told
me,
was
observed
by
them
for lactic
acid
production.
Another
point
which
has
appeared
in
every
curve
of
heat
production
obtained
in
this
paper,
is
that
over
the
time
during
which
the
heat
production
is
observed
(it
appears
in
Figs.
3
and
4),
equal
amounts
of
heat
are
not
obtained
in
equal
periods
of
stimulation,
approximately
800/0
of
the
heat
appears
in
the
first
2-3
minutes
of
stimulation.
In
the
curves
of
liberation
of
the
substances
causing
contraction
and
rigor
therefore,
there
seems
to
be
a
difference
between
liberation
leading
to
contraction
and
leading
to
rigor,
in
the
former
case
the
liberation
is
more
or
less
exponential,
in
the
latter
linear.
This
character
coupled
with
the
other
observations
in
the
paper
makes
it
probable
that
the
second
explanation
of
Fletcher
and
Hopkins'
for
the
fatigue
ratio
of
lactic
acid
is
the
true
one,
namely
that
the
"lactic
acid
of
fatigue
stops
short,
self-impeded
at
a
relatively
low
level."
The
exponential
character
of
the
heat
production
of
stimulation
would
admit
of
the
possibility
of
this
'self-impeding'
beingf
due
to
the
accumulation
of
the
substance
(?lactic
acid)
causing
contraction
in
a
small
place,
the
cessation
of
liberation
arriving
when
the
acid
had
reached
the
highest
concentration
possible
at
the
place
of
liberation.
In
the
end,
the
liberation
would
be
infinitely
slow.
A
simple
explanation
of
this
character,
however,
would
not
explain
the
splitting
off
of
acid
during
the
onset
of
rigor,
in
which
the
relation
is
a
linear
one.
In
this
con-
nection,
the
work
of
Embden
and
Kondo2
is
suggestive,
in
which
they
show
that
the
production
of
acid
seems
to
be
dependent
upon
the
hydrogen
ion
concentration
of
the
muscle
juice.
Mines
has
pointed
out
that
the
liberation
of
acid
probably
takes
place
in
extremely
localised
positions,
from
which
it
diffuses
away
into
the
general
substance
of
the
muscle,
or
is
removed
from
the
positions
of
action.
A
further
point
arising
from
this
paper,
which
needs
discussion,
is
whether
the
heat
which
has
been
measured
is
solely
a
heat
production
concerned
with
the
appearance
of
lactic
acid.
That
lactic
acid
is
the
main
body
appearing
in
the
process
of
contraction,
we
know
from
the
work
of
Fletcher
and
Hopkins;
and
therefore
that
the
heat
must
be
concerned
mainly
with
the
lactic
acid,
it
is
possible
to
say
with
a
fair
degree
of
certainty,
but
until
we
know
that
nothing
but
lactic
acid
1
Fletcher
and
Hop
kins.
This
Journal,
xxxv.
p.
281.
1907.
2
Biochem.
Zt8ch.
xiv.
p.
69.
1912.
269

0R
A.
PETERS.
appears
in
contraction
and
rigor
it
does
not
seem
possible
to
say
that
the
heat
is
concerned
solely
with
the
lactic
acid
that
appears.
Hill'
has
given
some
evidence
to
show
that
in
the
presence
of
oxygen
the
heat
production
for
short
periods
of
tetanus
occurs
in
two
stages.
If
we
accept
the
view
that
the
process
leading
to
contraction
occurs
in
two
stages,
one
the
breakdown
of
a
precursor
(A)
to
the
substance
(B)
causing
contraction,
the
other
the
removal
of
the
suib-
stance
(B)
causing
contraction
from
the
point
of
liberation,
the
two
stages
of
heat
production,
as
Hi
ll
suggests,
will
measure
(1)
A
-e
B
and
(2)
removal
of
B.
Stage
(2)
occurs
according
to
his
view
only
in
the
presence
of
oxygen.
If
the
experiments
in
this
paper
are
correct,
and
the
muscle
cannot
store
oxygen,
but
only
makes
a
contemporary
use
of
it
in
preparation
for
a
subsequent
contraction,
a
view
that
has
been
substantiated
by
the
experiments
of
VerzAr
upon
the
time
relations
of
the
oxygen
removal
from
the
blood
supplying
a
contracting
muscle
(as
mentioned
above)
it
would
seem
that
the
heat
production
measured
by
the
methods
of
this
paper
for
periods
of
contraction,
has
been
that
of
stage
(1)
only.
SUMMARY
AND
CONCLUSIONS.
With
a
view
to
testing
current
hypotheses
of
muscular
contraction,
from
the
standpoint
of
heat
production,
especially
as
to
whether
the
processes
of
contraction
and
rigor
call
upon
the
same
body
as
precursor,
Hill's
differential
calorimeter
bas
been
modified
for
the
purpose
of
measuring
the
heat
produced
during
the
indirect
stimulation
to
fatigue
of
frog's
limbs
through
the
sciatic
plexus.
The
conclusions
which
have
been
reached
are
that:
1.
The
heat
produced
during
the
indirect
stimulation
of
frog's
muscles
to
a
state
of
fatigue
in
atmospheric
air
has
a
maximum
value
approximating
to
0
9
cal.
per
gram
of
muscle
tissue.
The
heat
liberation
is
roughly
exponential,
about
70-80
0/0
being
liberated
in
the
first
2
mins.
of
tetanus.
2.
This
quantity
bears
a
ratio
of
about
one
half
to
the
heat
produced
in
the,
rigor
caused
by
the
poisoning
action
of
chloroform
upon
the
muscle
substance,
for
which
a
value
of
1'70
cals.
per
gram
is
obtained.
3.
The
heat
liberated
in
chloroform
rigor
induced
after
indirect
stimulation
to
fatigue
has
a
value
approximating
to
0-87
calorie
per
gram.
A.
V.
Hill.
This
Journal,
xLvI.
p.
28.
270

HEAT
PRODUCTION
OF
MUSCLE.
4.
Thus
the
sum
of
the
heat
produced
during
such
a
contraction,
and
the
heat
produced
after
it
by
chloroform
rigor,
is
approximately
equal
to
the
heat
production
of
chloroform
rigor
in
the
fresh
muscle.
This
result
confirms
the
argument,
from
the
lactic
acid
and
heat
production
in
rigor
and
contraction,
that
no
processes
of
importance
arise
in
the
production
of
rigor
other
than
are
involved
in
muscular
contraction.
5.
Estimations
of
the
lactic
acid
produced
under
the
experimental
conditions
of
the
heat
measurements
agree
with
those
of
Fletcher
and
Hopkins
for
fatigue
and
rigor.
Hence
the
heat
produiction
and
lactic-
acid
liberation
are
extremely
intimately
connected.
6.
By
exposing
the
muscle
to
pressures
of
one
atmosphere
of
oxygen
for
one
hour
before
the
experiment,
some
evidence
shows
that
the
heat
production
of
contraction
is
not
increased.
Besides
the
thanks
which
I
have
expressed
in
the
paper
I
should
like
to
express
my
warmest
gratitude
to
Mr
A.
V.
Hill
for
his
kind
help
throughout
this
research,
and
to
Dr
Fletcher
for
his
most
helpful
criticism.
(The
expenses
of
this
research
have
been
defrayed
in
part
by
a
grant
from
the
Thruston
Memorial
Fund,
Gonville
and
Caius
College,
and
in
part
by
a
grant
from
the
Royal
Society.)
271